
How to capture a malaria mosquito? Study and development of odour-baited traps
WIAS Magazine - Summer edition 2022
Research Highlight
In my PhD thesis, I aimed to contribute to our understanding of how haematophagous female mosquitoes interact with their hosts in order to get a protein-rich blood meal necessary for egg production. Many dangerous pathogens such as the malaria parasite and the dengue virus take advantage of this mosquito–vertebrate interaction to spread themselves. This is what arguably makes the most annoying animal in the world, and certainly the most dangerous animal in the world. Malaria alone kills more than 400,000 people – mostly young children – every year (WHO, 2020), and has killed many more in the past.
In order to get a blood meal, female mosquitoes must seek a vertebrate host (see figure 1). For this, they can detect CO2 and volatiles that signal the presence of a nearby host upwind (Cardé et al., 2010; Cardé, 2015). A flying mosquito will “surge” upwind in the direction of the odour source (Cardé and Willis, 2008; van Breugel and Dickinson, 2014). And if it loses the plume, the mosquito will “cast” crosswind to detect it again. Then, it will rely on visual cues to find the source of the odour plume (van Breugel et al., 2015; Vinauger et al., 2019). Up until this point, the host-seeking flight behaviour of mosquitoes has been studied extensively, most often in wind-tunnel experiments (Kennedy, 1940; Cooperband and Cardé, 2006; Spitzen et al., 2013; van Breugel et al., 2015; Hawkes and Gibson, 2016). Finally, the mosquito will use additional cues, so-called short-range cues such as heat and humidity, to decide whether and where it will land (Howlett, 1910; Beeuwkes et al., 2008; Hawkes and Gibson, 2016). The importance of these cues is also well established but the flight dynamics of mosquitoes and the role of airflow – another important cue used by mosquitoes – in close vicinity of their hosts is still relatively poorly understood.
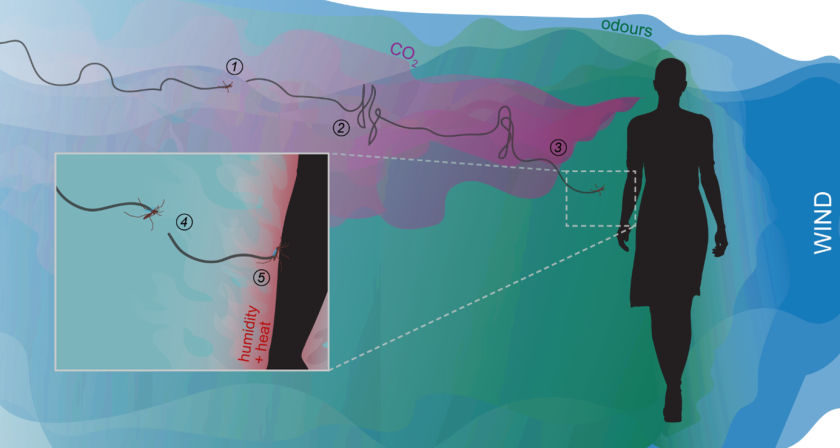
Figure 1. Infographic on how mosquitoes detect and fly towards a blood host. (1) A flying female mosquito encounters CO2 and odour plumes. (2) The mosquito will surge upwind and cast crosswind to find the source. (3) The mosquito will inspect visually interesting objects, and then (4) will use short-range host cues such as heat and humidity to select a spot to land (5). Figure from (Cribellier, 2021).
To capture mosquitoes, many different mosquito traps have been developed over the years (Kline, 2002; Kröckel et al., 2006; Jawara et al., 2009; Hiscox et al., 2014). A majority of these are aimed at capturing host-seeking female mosquitoes by mimicking human host cues (Kline, 2002; Kawada, Honda and Takagi, 2007; Bhalala and Arias, 2009). Such traps are called odour-baited traps because they use CO2 and odour blends to attract mosquitoes in their vicinity. These blends were developed based on the accumulated knowledge from many studies on mosquitoes attraction to CO2 and chemical compounds such as ammonia and lactic acid (Dekker, Geier and Cardé, 2005; Verhulst et al., 2009; Cardé, 2015; van Loon et al., 2015). Odour-baited traps often use counter-flows generated by a fan to diffuse the odour and CO2, thus attracting host seeking mosquitoes in their vicinity; and then capture mosquitoes that would have approached it by sucking them inside the trap (Kröckel et al., 2006; Hiscox et al., 2014). Until recently, odour-baited traps were exclusively used as tools for monitoring mosquito populations. However, they are now being considered as insecticide-free vector control tools that could effectively reduce mosquito populations when combined with bed nets (Hiscox et al., 2014; Homan et al., 2016).
In my first publication, we studied for the first time how mosquitoes behave around counter-flow odour-baited traps (Cribellier et al., 2018). We recorded thousands of three-dimensional flight tracks of female malaria mosquitoes (Anopheles coluzzii) around a well-known trap, the BG-Suna, in opposite orientations to represent two widely used traps. We visualized the average behaviour of mosquitoes and flight dynamics around the traps on two-dimensional heat maps. This allowed us to identify that mosquitoes were following stereotypical behaviours: When approaching the traps, by flying downward, and when close to the inlet of the traps, by accelerating upward. These behaviours led to very different capture dynamics of mosquitoes, and consequently to contrasting short-range attractiveness and capture mechanisms of the two traps. For example, the standing BG-Suna was more attractive than the hanging BG-Suna, while also being the only trap that triggered escape-like responses of mosquitoes.
Based on our findings from this first study, integrated with the literature, we developed a new counter-flow odour-baited trap: the M-Tego (see figure 2). One aspect of this trap is that it can generate two additional host cues that have been found to attract mosquitoes at short range, namely heat and humidity. In a second study, we tested this new trap against the BG-Suna in laboratory and semi-field conditions (Cribellier et al., 2020). In both conditions, the M-Tego without additional short-range cues was found to capture more than twice as many An. coluzzii than the standing BG-Suna. And when the M-Tego generated heat and humidity, it captured around 4.5 times as many mosquitoes as the BG-Suna. To understand why the M-Tego exhibited such improved capture rates, we recorded the flight tracks of mosquitoes around this new trap with or without additional short-range cues. Using similar analysis tools as in our first study, we showed that mosquitoes are more attracted to the M-Tego and spent more time close to it when the trap generates heat and/or humidity. Additionally, we did not observe escape-like responses of mosquitoes near the M-Tego, which seems to explains why the M-Tego captures more mosquitoes than the standing BG-Suna even when they produce the same host cues (CO2, odour and visual cues).
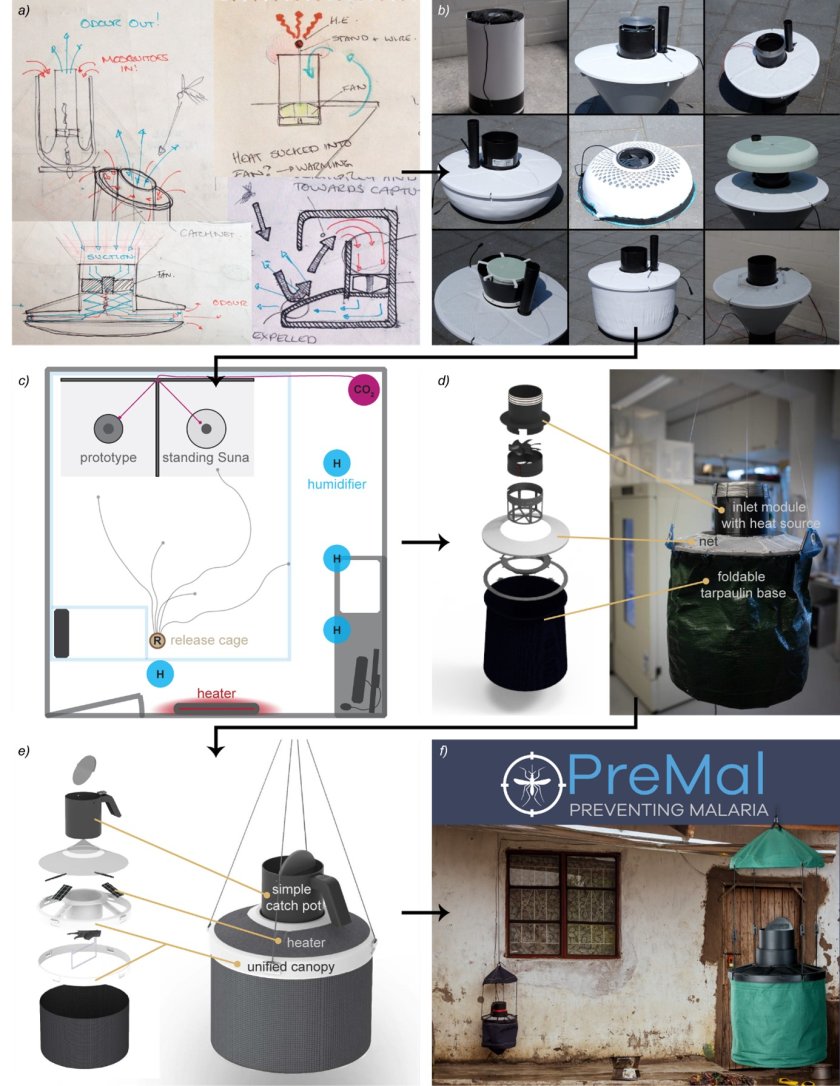
Figure 2. The development of the M-Tego. After discovering that counter-flow traps had low capture efficiency (Cribellier et al., 2018), the M-Tego trap was developed. (a) Many design ideas were put on paper. (b) Nine prototypes were built in order to test the most promising ideas. (c) The prototypes were tested against the standing Suna trap in dual-choice experiments. (d) A new trap, the M-Tego, was designed and a first prototype was built. This is the prototype that was tested in my second publication (Fairbairn, 2018; Cribellier et al., 2020). (e) A second version of the M-Tego was designed to make it easier to use and cheaper to build (van de Geer, 2019). (f) The final M-Tego was designed. It is now produced and distributed around the world by PreMal. Figure from (Cribellier, 2021).
Following this, PreMal BV, a start-up and spin-off from Wageningen University, was co-founded by Henry Fairbairn with whom I initially developed the trap. The M-Tego is now available in many regions around the world, and specifically in regions most affected by vector-borne diseases.
References:
Beeuwkes, J. et al. (2008) ‘3-D flight behaviour of the malaria mosquito Anopheles gambiae s . s . inside an odour plume’, Proceedings of the Netherlands Entomological Society Meeting, 19(November), pp. 137–146.
Bhalala, H. and Arias, J. R. (2009) ‘The Zumba mosquito trap and BG-Sentinel trap: Novel surveillance tools for host-seeking mosquitoes.’, Journal of the American Mosquito Control Association, 25(2), pp. 134–139. doi: 10.2987/08-5821.1.
van Breugel, F. et al. (2015) ‘Mosquitoes use vision to associate odor plumes with thermal targets’, Current Biology. Elsevier Ltd, 25(16), pp. 2123–2129. doi: 10.1016/j.cub.2015.06.046.
van Breugel, F. and Dickinson, M. H. (2014) ‘Plume-tracking behavior of flying drosophila emerges from a set of distinct sensory-motor reflexes’, Current Biology. Elsevier Ltd, 24(3), pp. 274–286. doi: 10.1016/j.cub.2013.12.023.
Cardé, R. T. et al. (2010) ‘Host finding by female mosquitoes : mechanisms of orientation to host odours and other cues’, in Olfaction in Vector-Host Interactions. Wageningen Academic Publishers, pp. 115–136.
Cardé, R. T. (2015) ‘Multi-cue integration: How female mosquitoes locate a human host’, Current Biology. Cambridge University Press, Cambridge, 25(18), pp. R793–R795. doi: 10.1016/j.cub.2015.07.057.
Cardé, R. T. and Willis, M. A. (2008) ‘Navigational strategies used by insects to find distant, wind-borne sources of odor’, Journal of Chemical Ecology. Springer-Verlag, 34(7), pp. 854–866. doi: 10.1007/s10886-008-9484-5.
Cooperband, M. F. and Cardé, R. T. (2006) ‘Comparison of plume structures of carbon dioxide emitted from different mosquito traps’, Medical and Veterinary Entomology. Blackwell Publishing Ltd, 20(1), pp. 1–10. doi: 10.1111/j.1365-2915.2006.00614.x.
Cribellier, A. et al. (2018) ‘Flight behaviour of malaria mosquitoes around odour-baited traps: Capture and escape dynamics’, Royal Society Open Science, 5(8). doi: 10.1098/rsos.180246.
Cribellier, A. et al. (2020) ‘Lure, retain, and catch malaria mosquitoes. How heat and humidity improve odour-baited trap performance’, Malaria Journal. BioMed Central, 19(1), pp. 1–16. doi: 10.1186/s12936-020-03403-5.
Cribellier, A. (2021) Biomechanics of flying mosquitoes during capture and escape. University of Wageningen. doi: 10.18174/552797.
Dekker, T., Geier, M. and Cardé, R. T. (2005) ‘Carbon dioxide instantly sensitizes female yellow fever mosquitoes to human skin odours’, Journal of Experimental Biology. The Company of Biologists Ltd, 208(15), pp. 2963–2972. doi: 10.1242/jeb.01736.
Fairbairn, H. (2018) Design of an Odour Baited Mosquito Trap for Malaria Prevention in Africa. University of Wageningen.
van de Geer, C. (2019) Adapting a mosquito trap for future deployment in African communities. University of Wageningen.
Hawkes, F. and Gibson, G. (2016) ‘Seeing is believing: the nocturnal malarial mosquito Anopheles coluzzii responds to visual host-cues when odour indicates a host is nearby.’, Parasites and Vectors. BioMed Central, 9, p. 320. doi: 10.1186/s13071-016-1609-z.
Hiscox, A. et al. (2014) ‘Development and optimization of the Suna trap as a tool for mosquito monitoring and control’, Malaria Journal, 13, p. 257. doi: 10.1186/1475-2875-13-257.
Homan, T. et al. (2016) ‘The effect of mass mosquito trapping on malaria transmission and disease burden (SolarMal): a stepped-wedge cluster-randomised trial’, The Lancet. Elsevier Ltd, 388(10050), pp. 1193–1201. doi: 10.1016/S0140-6736(16)30445-7.
Howlett, F. M. (1910) ‘The influence of temperature upon the biting of mosquitoes’, Parasitology. Cambridge University Press, 3(4), pp. 479–484. doi: 10.1017/S0031182000002304.
Jawara, M. et al. (2009) ‘Optimizing odor-baited trap methods for collecting mosquitoes during the malaria season in the Gambia’, PLoS ONE. Edited by G. Snounou. Public Library of Science, 4(12). doi: 10.1371/journal.pone.0008167.
Kawada, H., Honda, S. and Takagi, M. (2007) ‘Comparative laboratory study on the reaction of Aedes aegypti and Aedes albopictus to different attractive cues in a mosquito trap’, Journal of Medical Entomology, 44(3), pp. 427–432. doi: 10.1603/0022-2585(2007)44[427:CLSOTR]2.0.CO;2.
Kennedy, J. S. (1940) ‘The visual Responses of flying mosquitoes.’, Proceedings of the Zoological Society of London, 109 A(4), pp. 221–242. doi: 10.1111/j.1096-3642.1940.tb00831.x.
Kline, D. L. (2002) ‘Evaluation of various models of propane-powered mosquito traps.’, Journal of Vector Ecology, 27(1), pp. 1–7.
Kröckel, U. et al. (2006) ‘New tools for surveillance of adult yellow fever mosquitoes: Comparison of trap catches with human landing rates in an urban environment’, Journal of the American Mosquito Control Association, 22(2), pp. 229–238. doi: 10.2987/8756-971X(2006)22[229:NTFSOA]2.0.CO;2.
van Loon, J. J. J. A. et al. (2015) ‘Mosquito attraction: crucial role of carbon dioxide in formulation of a five-component blend of human-derived volatiles.’, Journal of Chemical Ecology. Springer US, 41(6), pp. 567–73. doi: 10.1007/s10886-015-0587-5.
Spitzen, J. et al. (2013) ‘A 3D analysis of flight behavior of Anopheles gambiae sensu stricto malaria mosquitoes in response to human odor and heat’, PLoS ONE, 8(5), pp. 1–12. doi: 10.1371/journal.pone.0062995.
Verhulst, N. O. et al. (2009) ‘Cultured skin microbiota attracts malaria mosquitoes’, Malaria Journal. BioMed Central, 8(1), pp. 1–12. doi: 10.1186/1475-2875-8-302.
Vinauger, C. et al. (2019) ‘Visual-olfactory integration in the human disease vector mosquito Aedes aegypti’, Current Biology. Cell Press, 29(15), pp. 2509-2516.e5. doi: 10.1016/j.cub.2019.06.043.
WHO (2020) World Malaria Report 2020, World Health Organization. WHO Press, Geneva.